Phytoremediation: plants are (always) good to us
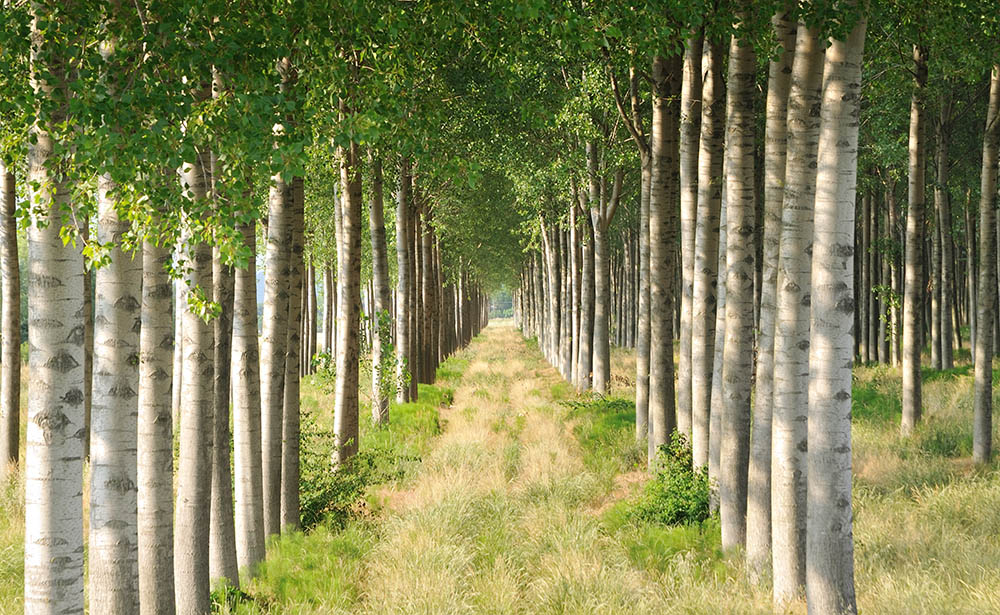
Since the beginning of industrialisation, humans have used the soil as a contaminant sink. Over time, the amount of pollutants disposed into soil has increased – landfills being a primary example of this1.
Soil is not a renewable resource and is teeming with ecosystems of micro-organisms that are vital to life on earth. Unfortunately, the ecosystems of soil are not as well-known as those found in water or air. Soil looks lifeless and static, but it provides us with services of inestimable value – our entire agricultural infrastructure critically depends upon soil1.
There are many pollutants that can cause harm to the equilibrium of a soil’s natural ecosystem, such as heavy metals, petroleum hydrocarbons, solvents, agrichemicals (amongst many others). Whilst some perceived pollutants (eg heavy metals) are naturally present in the soil from minerals, erosion and volcanic activity, the main and most dangerous source of pollution is the introduction of xenobiotic (human-made) pollutants through activities such as mining, smelting, utilising pesticides and fertilizer, sludge dumping and industrial discharging2.
So, how can we right the wrongs of our activities? Through remediation of contaminated land, – the removal of contaminants from the soil with a wide variety of techniques.
One of these techniques is the phytoremediation (from phyto meaning plant in Greek, and remedium meaning restoring balance in Latin), which uses plants to reduce the quantity or the toxicity of pollutants in the soil2.
Phytoremediation may sound like a new technology but the concept has been around since the 16th century. In the 16th century, Andrea Cesalpino realised that some plants could be grown on ultramafic soils (i.e. soils very rich in magnesium and iron) and, therefore, they were able to tolerate high concentrations of elements and compounds that are symptomatic of contaminated soils2. His research was forgotten and then rediscovered in the 1970s, when a scientist called Brooks named those plants ‘hyperaccumulators’2. In the meantime, other scientists found out that plants were able to absorb heavy metals such as nickel, but also capable of metabolising and thus removing from soil organic and dangerous compounds such as dichlorodiphenyltrichloroethane, better known as the pesticide DDT1.

Fig 1. Soil excavation
Conventional remediation methods include: soil incineration, excavation and disposal (aka ‘dig and dump’), soil washing, flushing and stabilization with electro-kinetic systems. All of these are characterised by high costs, intensive labour and irreversible changes to the soil, able to cause secondary problems and dangerous disturbance to the local ecological system2. By contrast, phytoremediation is easily implemented, involves minimal maintenance costs, and also enriches the soil with nutrients and organic matter from the plants – resulting in less contamination and natural fertilisation3. The contaminated land benefits from protection, root stabilisation, mitigation of erosion from water and wind in the form of vegetation cover. Moreover, there is no size restriction for the phytoremediation application, and the public perception generally considers it eco-friendly and more attractive than industrial procedures4.

Fig 2. Phytofiltration
Phytoremediation can be divided in different strategies2, 5:
- Phytoextraction: also known as phytoaccumulation, occurs when the plants extract the pollutants form the soil accumulating them in the above ground part of the plant.
- Phytofiltration: it is the process that involves removal of pollutants from surface water by roots or external parts of plants. In this instance, pollutants are retained in the soil and their movement is minimised (e.g. reed beds).
- Alternatively, phytostabilisation, phytovolatilisation and phytodegradation involve the chemical transformation of the contaminative compounds. It could involve pollutants in other environments changing their formula (for example in the atmosphere in a volatile form) or reducing their mobility and availability, thereby detoxifying them (e.g. breakdown of soil contaminants through metabolic processes within the plants).
The idea that plants can remove contaminants from soil has led to many studies aimed at using phytoremediation on a commercial scale2. Over the years phytoremediation has been tested, used and appreciated but also criticised. Currently, it is not the first option to employ as clean up solution, why is this?
Phytoremediation is limited by the hyperaccumulators’ slow growth, their low biomass, and their limited tolerance only to specific pollutants and not to a combination of them. Furthermore, the major limitation is the time; in fact, a long time is needed to make this technique effective, due to the slow absorption of small plants and the slow growth of trees2. Additionally, particular consideration needs to be given to the fate of the plants once they die, in order that the contaminants are not returned to the soil once the plant material decays.
So, the interest in phytoremendiation and with it the number of studies carried out about it is decreasing. Obstacles around the time taken for this remediation technique to be effective and the specific circumstances needed for hyperaccumulator plants to thrive (even they can’t grow in situations where the soil is particularly heavily contaminated) means that phytoremediation is not often economically viable. Additionally, in the laboratory tests used to assess if phytoremediation is applicable results can often be better than actual performances out in the field4 where new stressors not considered in the laboratory, can be encountered in the environment, resulting in the plants not thriving. Typical stressors are variation of temperature, precipitation, animals, plant pathogens, competition with weeds, and spatial variability of abiotic factors such as temperature, soil moisture and composition, or pH. Furthermore, the uptake of contaminants are limited to where the roots can grow (which means not beyond the 1 m depth, and in case of dendroremediation (phytoremediation using trees) it could reach 2 meters depth)4.

Fig. 3 Arboretum
Furthermore, adding non-native plants and their associated microbial fauna in new environments can lead to an intra-specific competition with the local microbial fauna, causing a loss of local biodiversity3. To combat this, using local plants, when possible, can also contribute to the reconstruction of the local ecosystem.
Much more remains to be studied about phytoremediation in order to encourage the use of this practice and overcome the challenges in its application. Phytoremediation should be considered at least as a complementary technology to be used along with the industrial techniques, in order to mitigate their negative impacts on the natural environment. New research and fieldwork would help to emphasize phytoremediation eco-friendly and sustainable qualities4, and possibly lead to discovering more sustainable remediation techniques. For instance, the number of studies about the capacity of mushrooms to break down contaminative pollutants through metabolic processes6 has been recently growing. Enhancing the importance and promoting awareness of low-cost bioremediation techniques could finally make of them the most opted options in terms or remediation.
References:
- Valentín, L., Nousiainen, A., & Mikkonen, A. (2012). Introduction to organic contaminants in soil: concepts and risks. In Emerging Organic Contaminants in Sludges (pp. 1-29). Springer Berlin Heidelberg.
- Ali, H., Khan, E., & Sajad, M. A. (2012). Phytoremediation of heavy metals—concepts and applications. Chemosphere, 91(7), 869-881.
- Cameselle, C., Chirakkara, R. A., & Reddy, K. R. (2012). Electrokinetic-enhanced phytoremediation of soils: status and opportunities. Chemosphere, 92(4), 626-626
- Gerhardt, K. E., Huang, X. D., Glick, B. R., & Greenberg, B. M. (2009). Phytoremediation and rhizoremediation of organic soil contaminants: potential and challenges. Plant Science, 176(1), 20-20.
- Raskin, I., Smith, R. D., & Salt, D. E. (1997). Phytoremediation of metals: using plants to remove pollutants from the environment. Current opinion in biotechnology, 8(2), 221-226
- EcoWatch, Researcher Develops Innovative Way to Use Fungi for Bioremediation of Oil-Contaminated Soil (2014) http://ecowatch.com/2014/05/23/mushrooms-fungi-bioremediation-contaminated-soil/
Date:
Apr 8, 2016
Author:
Eugenia Siccardi